New unpublished preclinical data has provided staggering new information on 1,3-butanediol and free acid BHB, showing that BHB supports increased liver ATP synthesis, while 1,3-butanediol (found in esters) is a massive liver ATP consumer during its conversion to BHB, thus depleting cellular energy.[1]
This article breaks down the new data, which shows how powerful BHB is, and adds growing concerns to the use of ketone esters like 1,3 butanediol in dietary supplements.
By now, we’ve all heard about the ketogenic diet. This diet, or way of eating, calls for restricting daily carbohydrate intake to roughly 50 grams or less, with a corresponding increase in fat consumption to replace the calories provided by carbs.[2]
Ketones: An Alternative Energy Source
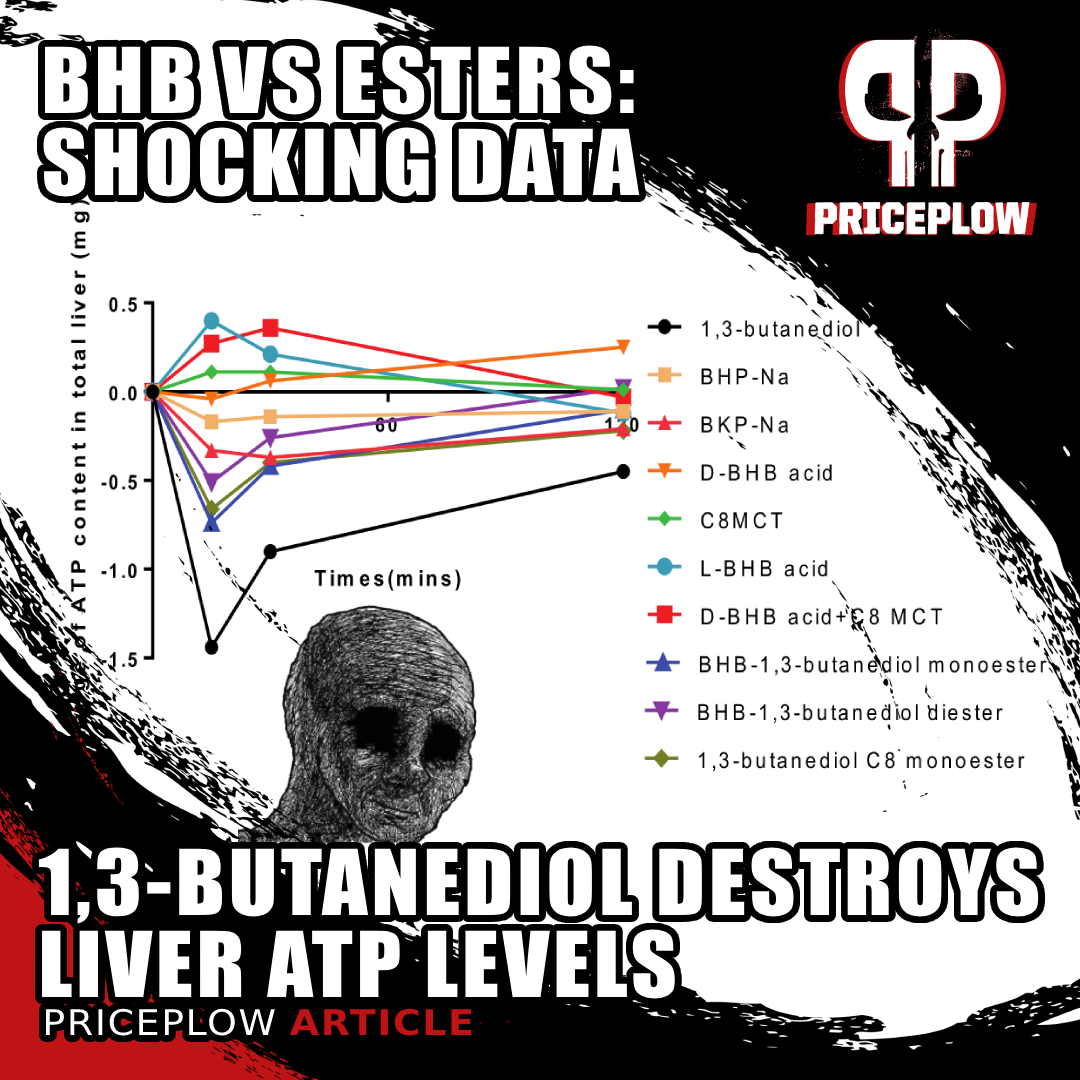
New unpublished preclinical data shows that 1,3-butanediol is a net ATP consumer in the liver, as opposed to free acid BHB and MCT which are ATP (energy) producers.[1] This sheds a whole new light on the BHB vs. Ketone Esters.
Once the body enters a state of ketosis, it breaks fat down into ketone bodies, which are used as an alternative energy source (as opposed to glucose).[2] This metabolic shift stabilizes blood sugar and insulin levels, which can be beneficial not only for preventing, but even for managing diabetes.[3]
Keto dieters commonly report enhanced mental clarity and focus, as relying on ketones for energy helps stabilize the brain’s metabolic supply. Many also report significantly decreased appetite and food cravings.[4] Therapeutically, ketogenic dieting shows promise in managing epilepsy, neurodegenerative diseases, and even certain cancers through its ability to impact key metabolic pathways and reduce inflammation.[5]
More controversially, some argue that keto can also improve long-distance athletic endurance, as the muscles’ adaptation to a consistent energy source helps prevent “bonking”, a point of failure reached by carbohydrate-reliant athletes who have depleted their glycogen reserves.[6,7]
Not just carbohydrate restriction: metabolic benefits of ketones themselves
One of the big questions is whether people see benefits from ketogenic diets due to the carbohydrate restriction, or instead from the benefits of the ketones themselves. Research shows that ketones improve cellular function by providing an efficient, stable energy source, reducing oxidative stress, and enhancing mitochondrial function.[8,9] They also have neuroprotective effects, and reduce neural inflammation.[10] This leads to improved overall cellular health and resilience, particularly in brain cells and muscle tissues (as shown in preclinical trials).[11-14]
Supporting ATP Production
The mechanism of action underlying all these profound benefits is ketones’ ability to serve as a substrate for the efficient production of ATP. Of course, glucose does too, but ketone-driven ATP synthesis is more efficient[15] and produces significantly fewer reactive oxygen species (ROS) than glucose-driven ATP synthesis, which explains most of ketone metabolism’s antioxidant effect, which is seen a fundamental in preventing premature cell death and dysfunction associated with aging.
It’s practically impossible to overstate the importance of having clean, efficient ATP synthesis. The analogy we use most frequently is that if your body were a car, ATP would be the gasoline. Every single action that every single one of your cells performs is completely dependent on ATP availability. Interruptions in fuel supply, or inefficiencies in burning it, can damage a car’s engine. Your body is no different – interruption in ATP supply can lead to cellular dysfunction, accumulation of toxic metabolites, and eventual cell death. If this cellular-metabolic damage gets bad enough, it has the potential to seriously compromise overall tissue and organ health.[17]
However, high-fat / low-carb ketogenic dieting (entering into a state of ketosis) isn’t the most practical or sustainable way to eat in our culture, and for that reason, keto diets require a lot of discipline and preparation to maintain. Fortunately, research shows that consuming exogenous ketones can offer much of the benefits associated with nutritional ketosis.
The question is, which type of ketone is best to work with?
In the past, we’ve covered the evolution of BHB salts and medium chain triglycerides (MCT oil, a ketone precursor which converts to BHB in the liver), and two podcasts (Episodes #131 and #138) exploring BHB free acid. But there are also various ketone precursors like 1,3-butanediol and ketone esters on the market. What works best with the fewest side effects, best taste, and least side effects?
New internal data from Ketone Labs, the trademark holders of goBHB and sponsor of this article, helps answer some of these questions:
BHB vs. 1,3-Butanediol – Which Exogenous Ketone Is Best?
Your body produces three different primary ketone bodies during nutritional ketosis:
Acetone, acetoacetic acid, and beta-hydroxybutyrate (BHB) are the three ketone bodies produced in the body. Image courtesy Wikipedia
- Beta-Hydroxybutyrate (BHB)
- Acetoacetate (AcAc)
- Acetone
Of these three, BHB is most commonly sold as an exogenous ketone supplement, for several reasons: it’s the most abundant ketone body in the blood during ketosis,[18] it’s the most chemically stable, making it easier to measure and more reliable for sustaining energy levels,[19] it’s the most metabolically efficient, and it provides more ATP molecules through oxidation than acetoacetate.[20,21]
BHB is also the easiest to formulate as a supplement – for example, it can be easily conjugated with various salts and esters, or sold as a free acid (great for liquid / beverage applications). It comes in two forms — L-BHB and D-BHB (they are also often combined), each isomer providing its own benefits.
However, BHB’s market dominance has been challenged lately by 1,3-butanediol. Unlike BHB, 1,3-butanediol is not a ketone body – it’s actually a direct precursor to BHB, meaning it must be metabolized by the liver to produce BHB.
Despite its horrific taste, 1,3-butanediol grew in popularity in the late 2010s when it was shown to dramatically increase blood BHB levels, often surpassing what consumers were getting with the mixed L/R BHB salts on the market at the time.
However, free acid BHB is now entering the market, and there’s some promising new unpublished research showing incredible efficacy and fewer metabolic consequences than the competition.
PricePlow Exclusive – BHB beats 1,3 Butanediol in New Animal Study
Direct comparisons of BHB against ketone esters like 1,3 butanediol are tough to come by. At the time of this writing, PricePlow is unable to locate a peer-reviewed, published, randomized controlled trial pitting this ketone ester against oral BHB.
Titled “The Impact of BHB Substrates and Precursors on Liver ATP”, this data showed a significant negative impact on liver ATP levels from 1,3-Butanediol.[1]
However, we have received exclusive access to a cutting-edge study that addresses this question – a study so new that it hasn’t even been published yet, so take it with a grain of salt.
Let’s dig into this study, and see what it can tell us about which of these two exogenous ketone supplements the typical consumer should opt for.
In this study, 32 mice were divided into 4 groups and administered a variety of ketone bodies and related compounds by means of intragastric gavage.[1] This is a fancy word for force-feeding – it’s a convenient way to standardize the oral consumption of an experimental treatment in animal study populations. From a physiological and metabolic perspective, there shouldn’t be any meaningful difference between this and ordinary oral consumption.
Here’s a complete list of the substances they administered:
- L-BHB
- D-BHB
- BHP-Na (Sodium β-hydroxypentanoate)
- BKP-Na (Sodium β-ketopentanoate)
- 1,3-butanediol
- C8 MCT (medium-chain triglycerides)
- D-BHB (80%) + C8 MCT (20%)
- BHB-1,3-butanediol monoester
- BHB-1,3-butanediol diester
- 1,3-butanediol C8 monoester
At the 0 minutes, 15 minute, 30 minute, and 120 minute (24 mice total) marks, the researchers measured the mice’s liver ATP production in response to each substance.[1]
Liver ATP – A critical measurement to understand
This study focused specifically on the liver because each of these ketone compounds has to undergo a series of conversion processes in the liver before they can become bioavailable as bloodborne ketone bodies. Since each compound will carry its own unique energy cost profile as it undergoes the conversion process, it stands to reason that some of them will generate more net ATP while being converted than others – and, in fact, some might even turn out to be net ATP consumers.
While ATP efficiency in the liver isn’t the whole picture of metabolic efficiency, it’s one very important part. In fact, hepatic ATP dynamics can have serious consequences for long-term metabolic health – one big example being the role that fructose can play in the onset of non-alcoholic fatty liver disease (NAFLD). Because a lot of ATP is hydrolyzed through the metabolism of fructose, it generates lots of adenosine di-phosphate (ADP) as a byproduct, which can then serve as a substrate for uric acid formation,[22] which is increasingly linked to the onset of NAFLD.[23]
Caution of unnecessary hepatic ATP consumers
In theory, all net hepatic ATP consumers, not just fructose, could contribute to fatty liver disease (hepatic steatosis) by depleting ATP reserves and increasing ADP levels. This depletion disrupts energy homeostasis, leading to an accumulation of AMP, which is then converted to uric acid.[22] Elevated uric acid levels cause oxidative stress and inflammation, further promoting liver damage.[24] Also, impaired ATP availability exacerbates insulin resistance and increases lipid accumulation in the liver.[25] These metabolic disturbances, oxidative stress, and inflammation collectively contribute to the development and progression of fatty liver disease.
So, while metabolic efficiency is important in general, it’s crucial in the liver. We want to consume net hepatic ATP producers, and avoid net hepatic ATP consumers, wherever possible.
With that in mind, we have to consider the results of this study as absolutely shocking: 1,3-Butanediol was a total disaster for hepatic cellular energy (ATP) production.[1]
As you can see from the measurements of liver ATP content at the various time points, the ketone ester absolutely hammered the liver from a cellular-metabolic standpoint.
Remember that in order to measure the total impact of a change over time, we want to take the area under the curve (AUC) of the function, also known as the definite integral in calculus. Basically, by taking the AUC of the graph showing changes in hepatic ATP content over time, we can measure the total change of hepatic ATP during the period of measurement.
So with that in mind, the results of this study get way more bleak when you look at a graph of each substance’s AUC:
If this study[1] is to be confirmed, 1,3-butanediol is what we consider to be straight-up liver poison.
The AUC analysis shows that compared to all the other ketones, 1,3-butanediol is a massive net consumer of ATP. Ultimately, all of the “precursor” esters are of concern, but 1,3-butanediol is far and away the worst.
1,3-Butanediol is a diol molecule — a functional alcohol!
This makes sense, because it is a diol molecule. The structural formula of 13B is as follows:
HO-CH₂-CH₂-CH(OH)-CH₃
The HO- and -OH groups in this molecule are functional alcohol groups.
This concerns us because alcohol consumption is a primary driver of hepatic steatosis (fatty liver), and the mechanism behind this association, as we discussed earlier, is net hepatic ATP consumption.
BHB is a net hepatic ATP producer
Conversely, the same graphs show quite clearly that BHB is a net hepatic ATP producer.[1] In other words, we expect that it would have the opposite effect – improving, rather than degrading the health and function of liver tissue.
As it stands, looking at this data, BHB (and MCT) are energy providers in the liver, while the precursor esters are energy depleters. If it takes you more ATP (energy currency) to produce something, it better be well worth it — and that simply doesn’t seem to be the case when we have alternatives in free acid BHB that do the opposite.
Once again, we must remind readers that this is not yet published data — it’s internally-produced. This article will be updated when that happens. But before concluding, we have to explore the published research on 1,3-butanediol:
Other side effects of 1,3-Butanediol
We hope the above data gets published, but it’s really only the final straw for 1,3-Butanediol. Looking into the published research, there are a lot of well-known side effects to consuming these ketone esters:[26-29]
- Body mass loss[26]
- Dehydration[26]
- Metabolic acidosis[26]
- Sinusoidal dilation[26]
- Stunted Growth[27]
- Hepatotoxicity[27,30]
- Decreased Fertility[28]
- Delayed fetal skeletal growth[28]
- Physical Dependence[29]
One study concludes with the following:[26]
“In summary, we report that 20% 1,3-BD, but not 5% or 10%, produces a systemic concentration of βHB similar to that observed after a 24-hour fast. However, this concentration is associated with deleterious side effects such as body mass loss, dehydration, metabolic acidosis, and sinusoidal dilation.”[26]
With or without this new ATP research, the benefits of some added BHB are simply not worth the consequences of the side effects listed above.
And it gets even worse:
Ketone Esters are used as alcohol-like beverages!
What’s more alarming is that some ketone esters are used as alcohol-like beverages! For instance, there are products known as “ketalcohol” or “ketohol” that utilize R-1,3-butanediol, which are marketed to provide a “real buzz”, despite having zero ethanol content.
If a ketone ester is used as a drink to give you a buzz… and it requires similar processing as alcohol… and it depletes liver ATP levels… should it be in dietary supplements?!
How does this happen? While BHB is generally considered safe and well-tolerated, the issue is that the metabolism of 1,3-butanediol is similar to that of ethanol![31] There’s a good chance that more than just BHB is being produced here, and we theorize that people are getting “buzzed” off of their metabolic byproducts.
Similarly, 1,3-butanediol has been reported to cause alcohol-like dependency issues.[29]
We won’t even get into 1,4-Butanediol, which is a whole different ballgame — the US Department of Justice has a document on its toxicity,[32] as it’s led to numerous overdoses, sometimes fatal.[33-35]
Ultimately, these secondary alcohols seem to be doing far more harm than good.
Conclusion: 1,3 Butanediol is a Metabolic Disaster
Beyond new energy drinks, there are tons of applications to consider with BHB, and formulators can now license goBHB to make their own
The supplement industry’s increasing use of 1,3-butanediol over BHB is, quite frankly, a grave mistake. Although it’s a natural BHB precursor, nobody knows the long-term effects of flooding the human body with potentially supraphysiological amounts of these esters, and in our opinion, until adequate safety studies are conducted on exogenous 13B, it should not be consumed if you care about ATP production (and everyone should care about ATP production).
Could regular 1,3-butanediol contribute to liver disease? What is the dose required to achieve this pathological effect? As always, it depends on the rest of your diet and current state of your liver, but ultimately, nobody finds out until it’s too late.
But given the potential for hepatic ATP depletion from 1,3-butanediol use, we think these questions deserve a research-based answer before the supplement industry puts it into common use. Until then, we have something more palatable that actually supports ATP production: Free Acid BHB.
You can sign up for our Ketone Labs and BHB news alerts below to get notified when new studies are published:
Comments and Discussion (Powered by the PricePlow Forum)